Week 1: Chemical and Biological Patterning
Many physical and chemical systems show spontaneous patterning, and creation of ordered structures from simple beginnings. You will explore some of these systems, and observe how processes of noise and feedback can drive the generation of patterned structures. While we’re using these as demonstrations of broader principles of self-organisation, the phenomena often have complicated underpinnings. Scientific reviews with more detailed explanations are provided at: https://haseloff.plantsci.cam.ac.uk/education/CDB_index/CDB_index.html. Note: Wear gloves and use protective eye wear for these experiments.
Collection of references that cover patterning systems.
Click here to download
Collection of references that cover patterning systems.
Click here to download
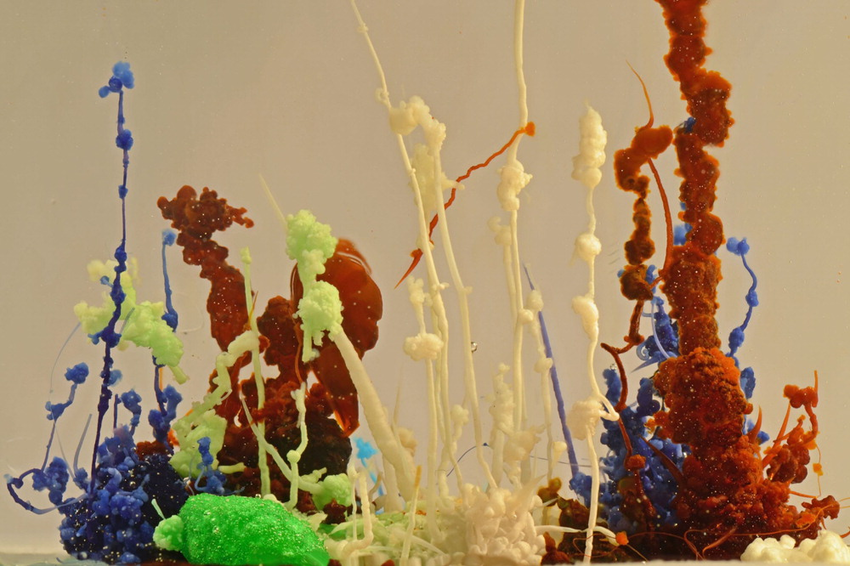
Chemical garden
Chemical gardens are an example in chemistry of a self-organising non-equilibrium process that creates complex structures. Many different chemical systems and materials can form these self-assembling structures, which span at least eight orders of magnitude in size, from nanometers to meters. Key to this marvel is the self-propagation under fluid advection of reaction zones forming semipermeable precipitation membranes that maintain steep concentration gradients, with osmosis and buoyancy as the driving forces for fluid flow.
EXPERIMENT 1: Chemical Garden 1. You are provided with a test tube (#1a) containing clear 30% sodium silicate solution (waterglass, 1.15 g/mL) and a tube (#1b) containing granule(s) soaked in metal salts. 2. Add the granule to the tube, place in a rack and leave undisturbed. 3. As you watch the tube, you should see the tube and needle-like structures spontaneously emerge from the metal salt containing granule.
Mechanism of growth of a classical chemical-garden structure from a metal-salt seed placed in sodium silicate solution (see figure above). (a) Setup at the start of the reaction. (b) Membrane formation between acidic and basic solutions. (c) Osmotic pressure is higher within the membrane than outside it, so the membrane expands. (d) Under osmotic forces, the membrane ruptures. (e) A tube forms. Hydroxide (OH–) is preferentially drawn into the interior of the membrane. The ionic and pH gradients across the membrane give rise to a membrane potential, as there is a difference in charge between the inner and outer solutions.
Reference: Formation of chemical gardens, Cartwright et al.,
Chemical gardens are an example in chemistry of a self-organising non-equilibrium process that creates complex structures. Many different chemical systems and materials can form these self-assembling structures, which span at least eight orders of magnitude in size, from nanometers to meters. Key to this marvel is the self-propagation under fluid advection of reaction zones forming semipermeable precipitation membranes that maintain steep concentration gradients, with osmosis and buoyancy as the driving forces for fluid flow.
EXPERIMENT 1: Chemical Garden 1. You are provided with a test tube (#1a) containing clear 30% sodium silicate solution (waterglass, 1.15 g/mL) and a tube (#1b) containing granule(s) soaked in metal salts. 2. Add the granule to the tube, place in a rack and leave undisturbed. 3. As you watch the tube, you should see the tube and needle-like structures spontaneously emerge from the metal salt containing granule.
Mechanism of growth of a classical chemical-garden structure from a metal-salt seed placed in sodium silicate solution (see figure above). (a) Setup at the start of the reaction. (b) Membrane formation between acidic and basic solutions. (c) Osmotic pressure is higher within the membrane than outside it, so the membrane expands. (d) Under osmotic forces, the membrane ruptures. (e) A tube forms. Hydroxide (OH–) is preferentially drawn into the interior of the membrane. The ionic and pH gradients across the membrane give rise to a membrane potential, as there is a difference in charge between the inner and outer solutions.
Reference: Formation of chemical gardens, Cartwright et al.,
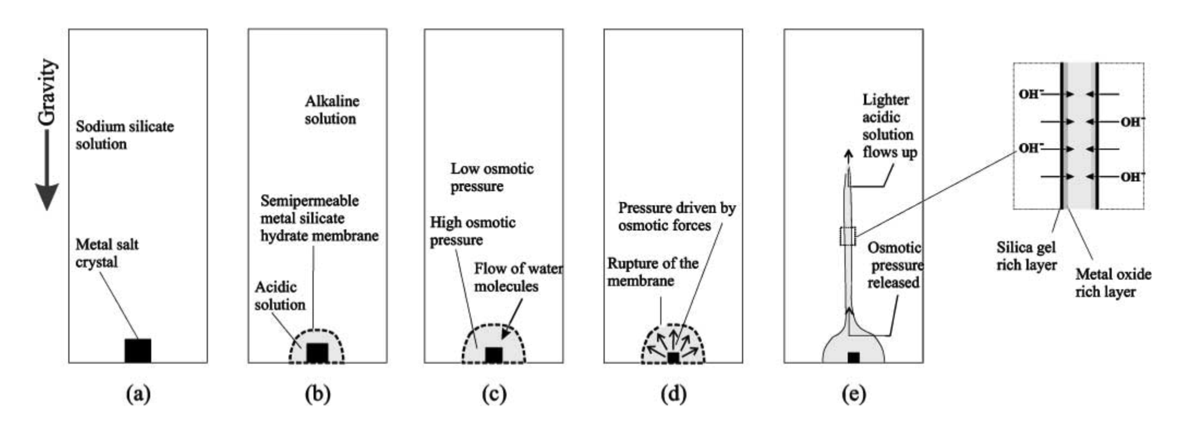
Lorem ipsum dolor sit amet, sapien platea morbi dolor lacus nunc, nunc ullamcorper. Felis aliquet egestas vitae, nibh ante quis quis dolor sed mauris. Erat lectus sem ut lobortis, adipiscing ligula eleifend, sodales fringilla mattis dui nullam. Ac massa aliquet.
Liesgang precipitation rings
Liesegang patterning is a special type of chemical pattern formation in which the spatial order is formed by density fluctuations of a weakly soluble salt. From analytical chemistry we know many different reactants that form a precipitate (sparingly soluble salt) when they react with each other. Coupling between precipitation kinetics and reactant transport can produce ordered patterns and self-organize complex structures. Liesegang patterns are produced in a simple experimental setup: an aqueous solution of an “outer” electrolyte is layered onto a gel containing its complementary “in- ner” electrolyte. Specifically, the diffusion of the outer electrolyte into the reaction medium induces the formation of regularly spaced precipitate aggregates. These patterns are periodic bands in one spatial dimension. Liesegang patterns have been observed for a broad range of systems involving metals (such as Ag+, Pb2+, Fe2+, Zn2+, Ca2+, and Mg2+) and their precipitate counter-anions (I−, F−, S2−, OH−, CrO42−, Cr2O72−, and SO42−), even extending beyond the laboratory to geochemical and mineralogical environments. The precise mechanism for this superficially simple phenomenon is unknown. A number of theories have been proposed (http://www.insilico.hu/liesegang/theories/theories.html) - all invoke some kind of autocatalytic or autoinhibitory process - such as alternating rounds of diffusion and “explosion” of supersaturated or coagulated precipitation. EXPERIMENT 2: Liesgang Rings 1. You are provided with a test tube (#2a) that contains 0.15 M copper sulphate evenly distributed in a swollen and polymerised gel (gelatine) - this is light blue coloured. 2. Label this tube with your names. 3. A second tube (#2b) contains a clear 0.1M solution of silver nitrate (AgNO3), pour this over the solid contents of tube #2a. Seal the overlaid tube and leave in an undisturbed place. 4. You should see the beginning of the formation of a precipitation band at the top of the polymerised gel. Leave the tube to observe in Week 2. The periodic precipitation reaction depends on the slow diffusion of the silver ions into the gel.
Liesegang patterning is a special type of chemical pattern formation in which the spatial order is formed by density fluctuations of a weakly soluble salt. From analytical chemistry we know many different reactants that form a precipitate (sparingly soluble salt) when they react with each other. Coupling between precipitation kinetics and reactant transport can produce ordered patterns and self-organize complex structures. Liesegang patterns are produced in a simple experimental setup: an aqueous solution of an “outer” electrolyte is layered onto a gel containing its complementary “in- ner” electrolyte. Specifically, the diffusion of the outer electrolyte into the reaction medium induces the formation of regularly spaced precipitate aggregates. These patterns are periodic bands in one spatial dimension. Liesegang patterns have been observed for a broad range of systems involving metals (such as Ag+, Pb2+, Fe2+, Zn2+, Ca2+, and Mg2+) and their precipitate counter-anions (I−, F−, S2−, OH−, CrO42−, Cr2O72−, and SO42−), even extending beyond the laboratory to geochemical and mineralogical environments. The precise mechanism for this superficially simple phenomenon is unknown. A number of theories have been proposed (http://www.insilico.hu/liesegang/theories/theories.html) - all invoke some kind of autocatalytic or autoinhibitory process - such as alternating rounds of diffusion and “explosion” of supersaturated or coagulated precipitation. EXPERIMENT 2: Liesgang Rings 1. You are provided with a test tube (#2a) that contains 0.15 M copper sulphate evenly distributed in a swollen and polymerised gel (gelatine) - this is light blue coloured. 2. Label this tube with your names. 3. A second tube (#2b) contains a clear 0.1M solution of silver nitrate (AgNO3), pour this over the solid contents of tube #2a. Seal the overlaid tube and leave in an undisturbed place. 4. You should see the beginning of the formation of a precipitation band at the top of the polymerised gel. Leave the tube to observe in Week 2. The periodic precipitation reaction depends on the slow diffusion of the silver ions into the gel.
Cell-like precipitation patterns
Reaction-diffusion systems where one of the reagents outer electrolyte penetrates into a gel by diffusion and forms a precipitate with the other reagent inner electrolyte homogenized in the gel, are able to produce various complex precipitation patterns. The mixing of CuCl2 and K3 Fe(CN)6, where the first reagent is the outer electrolyte and the other is the inner electrolyte homogenised in a gel is able to spontaneously generate tessellations of a plane through regressing edges of multiple reaction fronts. The spontaneous partitioning of the reaction results in the construction of a pattern analogous to a field of cells or a Voronoi diagram. EXPERIMENT 3: Voronoi precipitation reactions 1. You will be provided with a small Petri dish (#3a) containing 0.15M K3[Fe(CN)6] in 2% w/v agarose gel. 2. Locate tube (#3b) containing 2.9M CuCl2, and pour the contents over the top of the plate #3a, quickly and evenly. 3. You should see the immediate formation of a dark precipitate over the surface of the gel. You will see the spontaneous formation of “cell”-like centres and boundaries. 4. After the reaction has progressed, you may tip off the upper copper solution, and examine the surface of the plate under a low power (4x magnification) microscopy objective, to examine the features in more detail.
Schematic representation of Voronoi diagram formation through the ‘‘regressing edge mechanism.’’ (1) Outer electrolyte, (2) agarose gel containing the inner electrolyte, (3) precipitate, (4) reaction front active border, represented by a dotted surface, (5) passive border, (6) regressing edge. The contours of the patterns are drawn on the bottom of the vessel. A. The precipitation starts spontaneously at some points of the gel surface. B. These points expand into precipitate-free cones as the front progresses. C. The regressing edges meet on the line that is an equal distance from the tip of the empty cones.
Reaction-diffusion systems where one of the reagents outer electrolyte penetrates into a gel by diffusion and forms a precipitate with the other reagent inner electrolyte homogenized in the gel, are able to produce various complex precipitation patterns. The mixing of CuCl2 and K3 Fe(CN)6, where the first reagent is the outer electrolyte and the other is the inner electrolyte homogenised in a gel is able to spontaneously generate tessellations of a plane through regressing edges of multiple reaction fronts. The spontaneous partitioning of the reaction results in the construction of a pattern analogous to a field of cells or a Voronoi diagram. EXPERIMENT 3: Voronoi precipitation reactions 1. You will be provided with a small Petri dish (#3a) containing 0.15M K3[Fe(CN)6] in 2% w/v agarose gel. 2. Locate tube (#3b) containing 2.9M CuCl2, and pour the contents over the top of the plate #3a, quickly and evenly. 3. You should see the immediate formation of a dark precipitate over the surface of the gel. You will see the spontaneous formation of “cell”-like centres and boundaries. 4. After the reaction has progressed, you may tip off the upper copper solution, and examine the surface of the plate under a low power (4x magnification) microscopy objective, to examine the features in more detail.
Schematic representation of Voronoi diagram formation through the ‘‘regressing edge mechanism.’’ (1) Outer electrolyte, (2) agarose gel containing the inner electrolyte, (3) precipitate, (4) reaction front active border, represented by a dotted surface, (5) passive border, (6) regressing edge. The contours of the patterns are drawn on the bottom of the vessel. A. The precipitation starts spontaneously at some points of the gel surface. B. These points expand into precipitate-free cones as the front progresses. C. The regressing edges meet on the line that is an equal distance from the tip of the empty cones.
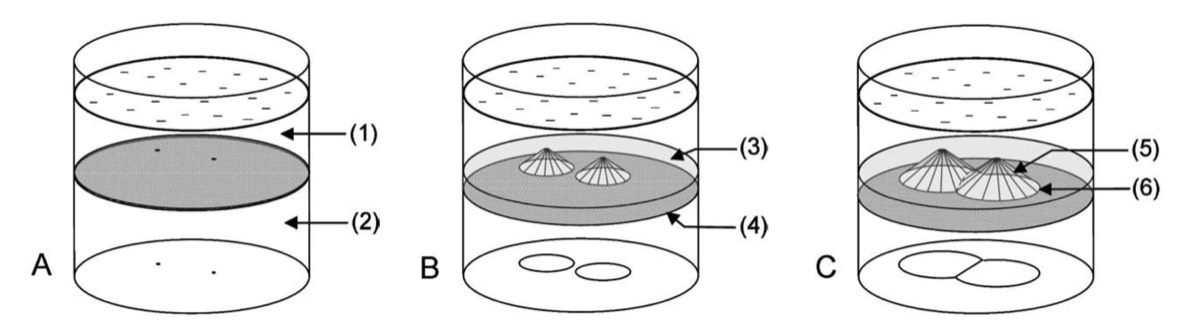
Belousov-Zhabotinsky reactions
The Belousov-Zhabotinsky (BZ) reaction is a chemical process that can show temporal oscillatory behavior or spatio-temporal structures as propagating waves, using a `bromate' (e.g., sodium bromate), an acid (e.g., sulfuric acid), a one electron redox catalyst/indicator (e.g., ferroin), and an organic component (e.g., malonic acid). Boris P. Belousov discovered a form of this reaction in 1950 in Moscow. He was unable to publish his results due to a general belief that his reaction violated the 2nd Law of Thermodynamics. The original work was not published until 1981. Anatol Zhabotinsky, as a student enrolled in Moscow State University became interested in oscillating chemical reactions and heard about Belousov's discovery and attempted to meet Belousov. Due to Belousov's classified work in countering the effects of radiation and chemical weapons, Belousov was reluctant to meet with Zhabotinsky but did confirm the recipe for the BZ-Reaction to Zhabotinsky over the phone and through mail. Zhabotinsky then worked on, and refined the chemical recipes. Zhabotinsky was successful in publishing the his results in a biochemistry journal, rather than a chemistry journal. In 1968, Zhabotinsky presented his work at the Conference on Biological and Biochemical Oscillators in Prague, Czechoslovakia, bringing worldwide attention to the reaction.
The BZ reaction, serves as a classical example of non-equilibrium thermodynamics, resulting in the establishment of a nonlinear chemical oscillator. It shows that chemical reactions do not have to be dominated by equilibrium thermodynamic behavior. These reactions are far from equilibrium and remain so for a significant length of time and evolve chaotically. An essential aspect of the BZ reaction is its so called "excitability"; under the influence of stimuli, patterns develop in what would otherwise be a perfectly quiescent medium.
The Belousov-Zhabotinsky (BZ) reaction is a chemical process that can show temporal oscillatory behavior or spatio-temporal structures as propagating waves, using a `bromate' (e.g., sodium bromate), an acid (e.g., sulfuric acid), a one electron redox catalyst/indicator (e.g., ferroin), and an organic component (e.g., malonic acid). Boris P. Belousov discovered a form of this reaction in 1950 in Moscow. He was unable to publish his results due to a general belief that his reaction violated the 2nd Law of Thermodynamics. The original work was not published until 1981. Anatol Zhabotinsky, as a student enrolled in Moscow State University became interested in oscillating chemical reactions and heard about Belousov's discovery and attempted to meet Belousov. Due to Belousov's classified work in countering the effects of radiation and chemical weapons, Belousov was reluctant to meet with Zhabotinsky but did confirm the recipe for the BZ-Reaction to Zhabotinsky over the phone and through mail. Zhabotinsky then worked on, and refined the chemical recipes. Zhabotinsky was successful in publishing the his results in a biochemistry journal, rather than a chemistry journal. In 1968, Zhabotinsky presented his work at the Conference on Biological and Biochemical Oscillators in Prague, Czechoslovakia, bringing worldwide attention to the reaction.
The BZ reaction, serves as a classical example of non-equilibrium thermodynamics, resulting in the establishment of a nonlinear chemical oscillator. It shows that chemical reactions do not have to be dominated by equilibrium thermodynamic behavior. These reactions are far from equilibrium and remain so for a significant length of time and evolve chaotically. An essential aspect of the BZ reaction is its so called "excitability"; under the influence of stimuli, patterns develop in what would otherwise be a perfectly quiescent medium.
EXPERIMENT 4: Belousov-Zhabotinsky reaction
1. You will be provided with aliquots of the components of the BZ reaction: (#4a) Acidified sodium bromate (0.5M NaBrO3, 0.5M H2SO4, (#4b) Acidified malonic acid (0.4M CH2(COOH)2, 0.5M H2SO4, plus 0.1% (w/v) SDS detergent (#4c) Solution of ferroin, a redox indicator (25mM Fe (1,10-phenanthroline)3 SO4)
2. Mix the reactants in a 5 cm diameter Petri dish. Gently swirl the plate to ensure mixing of the reactants. Ferroin is a bright red colour. After mixing the solution should immediately turn a bright blue colour, followed by darkened state.
3. The solution should be left undisturbed, preferably on a white coloured surface. The solution will eventually turn again to a red colour. If the solution is held in a thin layer, points within the solution will be seen to form in blue tinted relief from the rest of the red solution. If left to develop undisturbed, these points will form travelling waves, where the reaction behaves as an excitable medium. Patterns should develop spontaneously over the course of and hour or two - even reforming if the solution is disturbed.
1. You will be provided with aliquots of the components of the BZ reaction: (#4a) Acidified sodium bromate (0.5M NaBrO3, 0.5M H2SO4, (#4b) Acidified malonic acid (0.4M CH2(COOH)2, 0.5M H2SO4, plus 0.1% (w/v) SDS detergent (#4c) Solution of ferroin, a redox indicator (25mM Fe (1,10-phenanthroline)3 SO4)
2. Mix the reactants in a 5 cm diameter Petri dish. Gently swirl the plate to ensure mixing of the reactants. Ferroin is a bright red colour. After mixing the solution should immediately turn a bright blue colour, followed by darkened state.
3. The solution should be left undisturbed, preferably on a white coloured surface. The solution will eventually turn again to a red colour. If the solution is held in a thin layer, points within the solution will be seen to form in blue tinted relief from the rest of the red solution. If left to develop undisturbed, these points will form travelling waves, where the reaction behaves as an excitable medium. Patterns should develop spontaneously over the course of and hour or two - even reforming if the solution is disturbed.
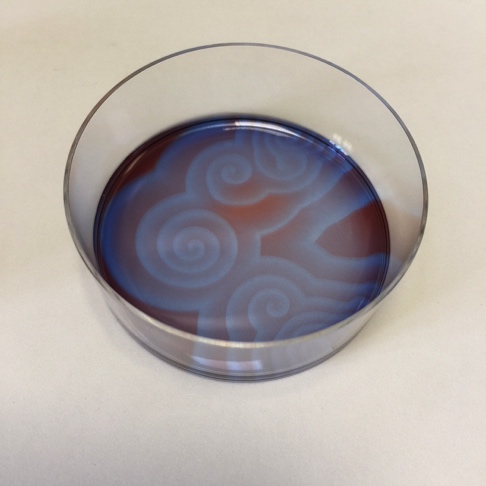
The BZ reaction mechanism is highly complex, with many (~50) individual reactions that fall into several categories and involve complex interplay of many inorganic and organic molecules. The oscillations in this extremely complex system arise due to the interplay of autocatalytic positive feedback and time delayed negative feedback. In the BZ reaction, the concentration of bromous acid rises sharply when it exceeds a threshold dependent upon the concentration of a second chemical species, bromide ions; the rising bromous acid concentration then causes bromide ions to be produced, which subsequently cause the bromous acid concentration to fall. Waves propagate when a high bromous acid concentration in one area spreads to adjacent areas and causes the bromous acid concentration to rise above threshold there. It is of considerable interest because of similarities to patterning, self-organisation and signal propagation in biological systems. For example, the wave behaviour of the BZ reactions is analogous to organisation of high membrane potentials on the surface of a beating heart, and many biological systems behave as excitable media.
A relevant extract from Phillip Ball’s book “Shapes - Nature’s Patterns: a tapestry in three parts” is provided with other online reference material. This contains a non-technical description of the processes at work in this complex set of reactions.
A relevant extract from Phillip Ball’s book “Shapes - Nature’s Patterns: a tapestry in three parts” is provided with other online reference material. This contains a non-technical description of the processes at work in this complex set of reactions.
Designing a test for cellular patterning in plant tissues
The selective traffic of auxin regulates cell polarity in plant tissues. A combination of influx and highly selective efflux transporters coordinate the flow of auxin within the plant and control cell fates and outgrowth. Further, auxin regulates the flux of its own traffic through plant tissues. Apical-basal polarity in plant embryos, outgrowth of the root and shoot meristem and initiation and maintenance of the plant vascular system are all dependent on this precise feedback-regulated traffic of auxin.
Current models for auxin based regulation of plant cell growth and differentiation rely on a “bucket brigade” style polar traffic of auxin, via specifically localised efflux carriers throughout the plant. Some form of positive feedback between efflux carrier and auxin flux results in canalisation of long-distance pathways for auxin traffic. The same mechanism also provides a route for local control of cell fates through cellular responses to auxin.
Cross-talk between auxin and other phytohormones such as cytokinin play a major role in the overall patterning of plant form. Cytokinin production and response are associated with cell division and growing points in plant tissue. Plants generally retain groups of stem cells in “meristems”, and these remain functional throughout the life of the plant and contribute to growth of the mature form.
Current models for auxin based regulation of plant cell growth and differentiation rely on a “bucket brigade” style polar traffic of auxin, via specifically localised efflux carriers throughout the plant. Some form of positive feedback between efflux carrier and auxin flux results in canalisation of long-distance pathways for auxin traffic. The same mechanism also provides a route for local control of cell fates through cellular responses to auxin.
Cross-talk between auxin and other phytohormones such as cytokinin play a major role in the overall patterning of plant form. Cytokinin production and response are associated with cell division and growing points in plant tissue. Plants generally retain groups of stem cells in “meristems”, and these remain functional throughout the life of the plant and contribute to growth of the mature form.
Marchantia polymorpha
In this practical, we introduce the simple model plant Marchantia polymorpha. Marchantia is the best-characterised liverwort, member of a class of Bryophytes that diverged from higher plants about 500 million years ago. Its ease of use, open form of development, haploid genetics and reduced gene set have generated great interest in its use as a new model plant. You can find a general introduction to the Marchantia system on the CDB Practical web pages at https://haseloff.plantsci.cam.ac.uk/education/CDB_index/CDB_index.html
One of the features of the Marchantia system is that it naturally produces prolific vegetative propagules, named gemmae, that can he harvested and grown in sterile culture. Germination and subsequent development occur in the open, and can be monitored directly by microscopy.
Some salient facts are:
Gemmae are produced within cup-like structures (“splash cups”) on the surface of the parent plant. The propagules are typically spread by water splash. As they land on a suitable substrate, they must quickly establish the correct dorsal-ventral polarity. Light and gravity provide cues for this, and auxin response is also required.
Day 1: Gemmae germinate immediately, and grow in stereotypical and staged fashion. Cells at apical notches on both ends of the gemmae undergo division. Cells in the central portion of the gemmae do not divide, but expand. The gemmae possess mirror symmetry at this stage.
Day 2: Long root hair-like rhizoids form, sometimes initially on both ventral and dorsal surfaces, but are eventually limited to the ventral side of the growing plant. Oil cells may also be apparent on the dorsal surface.
Day 4-5 The meristematic notches remain active, and distal cells under differentiation to produce air chambers. These can be recognised by characteristic air pore cell complexes. These will continue to form over the life of the plant and are specialised structures for photosynthesis and gas exchange.
Week 1: Secondary meristematic regions can result in the formation of additional “flaps” of tissue around the meristematic notches, as growth continues.
Week 2: First appearance of splash cups, the conical cup-like structures that produce new gemmae from a lower somatic embryogenic layer.
These timings are approximate, depending on growth conditions. However, the order and relative locations of differentiated features is relatively constant. Marchantia tissues have an extremely high capacity for regeneration, and tolerate surgical disruption. Unlike chemical systems, that are driven by physical processes, biological patterning is driven by genetic and cellular signalling and feedback. Removal of tissues that normally provide endogenous sources of phytohormone can disrupt in vivo feedback circuits and reset patterns of development.
Your challenge is to design an experiment to investigate the roles of auxin and cytokinin in growth and development of this simple plant system.
You have also been given a sterile scalpel, and other instruments that can be sterilised with ethanol when needed. How can you use these materials to test the roles of auxin and cytokinin in growth of Marchantia gemmae?
In this practical, we introduce the simple model plant Marchantia polymorpha. Marchantia is the best-characterised liverwort, member of a class of Bryophytes that diverged from higher plants about 500 million years ago. Its ease of use, open form of development, haploid genetics and reduced gene set have generated great interest in its use as a new model plant. You can find a general introduction to the Marchantia system on the CDB Practical web pages at https://haseloff.plantsci.cam.ac.uk/education/CDB_index/CDB_index.html
One of the features of the Marchantia system is that it naturally produces prolific vegetative propagules, named gemmae, that can he harvested and grown in sterile culture. Germination and subsequent development occur in the open, and can be monitored directly by microscopy.
Some salient facts are:
Gemmae are produced within cup-like structures (“splash cups”) on the surface of the parent plant. The propagules are typically spread by water splash. As they land on a suitable substrate, they must quickly establish the correct dorsal-ventral polarity. Light and gravity provide cues for this, and auxin response is also required.
Day 1: Gemmae germinate immediately, and grow in stereotypical and staged fashion. Cells at apical notches on both ends of the gemmae undergo division. Cells in the central portion of the gemmae do not divide, but expand. The gemmae possess mirror symmetry at this stage.
Day 2: Long root hair-like rhizoids form, sometimes initially on both ventral and dorsal surfaces, but are eventually limited to the ventral side of the growing plant. Oil cells may also be apparent on the dorsal surface.
Day 4-5 The meristematic notches remain active, and distal cells under differentiation to produce air chambers. These can be recognised by characteristic air pore cell complexes. These will continue to form over the life of the plant and are specialised structures for photosynthesis and gas exchange.
Week 1: Secondary meristematic regions can result in the formation of additional “flaps” of tissue around the meristematic notches, as growth continues.
Week 2: First appearance of splash cups, the conical cup-like structures that produce new gemmae from a lower somatic embryogenic layer.
These timings are approximate, depending on growth conditions. However, the order and relative locations of differentiated features is relatively constant. Marchantia tissues have an extremely high capacity for regeneration, and tolerate surgical disruption. Unlike chemical systems, that are driven by physical processes, biological patterning is driven by genetic and cellular signalling and feedback. Removal of tissues that normally provide endogenous sources of phytohormone can disrupt in vivo feedback circuits and reset patterns of development.
Your challenge is to design an experiment to investigate the roles of auxin and cytokinin in growth and development of this simple plant system.
You have also been given a sterile scalpel, and other instruments that can be sterilised with ethanol when needed. How can you use these materials to test the roles of auxin and cytokinin in growth of Marchantia gemmae?
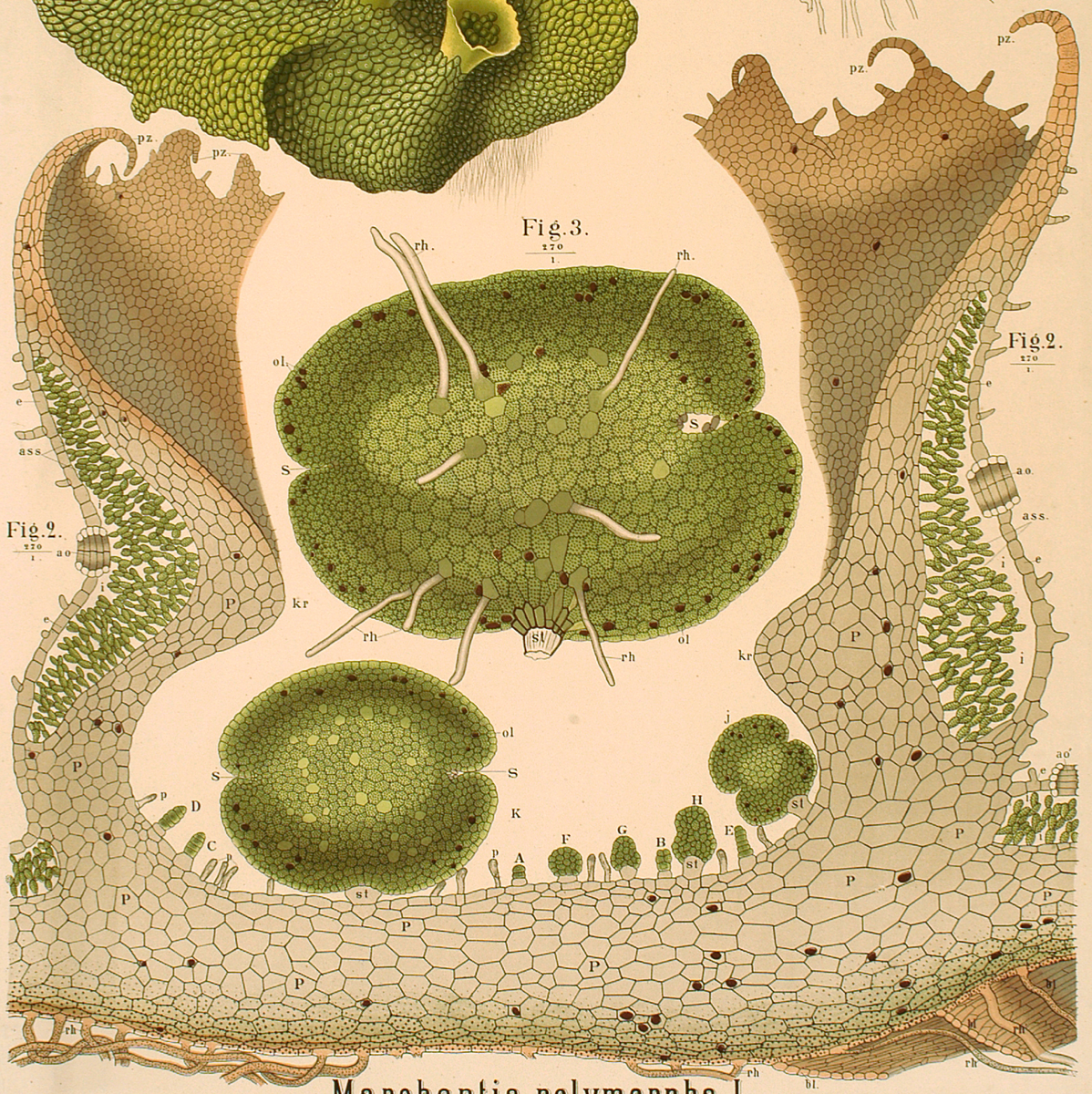
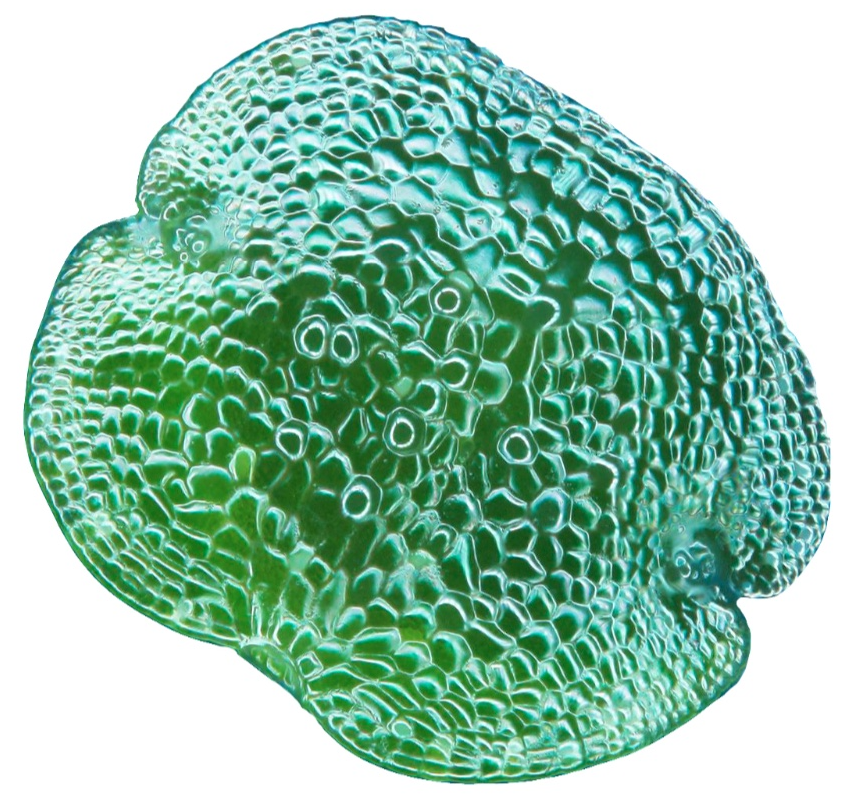
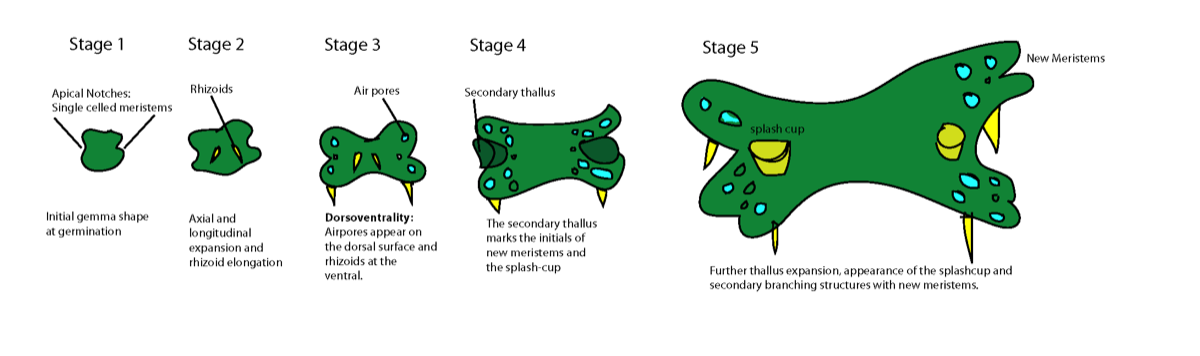
EXPERIMENT 5: Design an experimental protocol
Test for phytohormone regulation of gemmae growth
1. You are provided with an agar plate containing Marchantia gemmae that have been growing in sterile media for about one week.
2. Inspect the gemmae in the sealed plate, using a compound microscope and low power (4x) objective. Identify key features on the gemmae: meristematic regions, central portion, rhizoids, oil cells (if present), air pores and chambers.
3. What are the main recognisable features (and possible functional domains) of the gemmae? Auxin has been long implicated in establishment of polarity and cell fate in higher plants. Cytokinins have been implicated in meristem maintenance and antagonism to auxin. How might you disrupt interactions across the young plantlets? Do you have any candidate roles for auxin and cytokinin? What might you look for, if you disrupt and re-plate gemmae? Can you probe the role of auxin and cytokinin in cell proliferation versus patterning and differentiation?
4. The gemmae are maintained in sterile culture, so any manipulations, including transfer to new plates, should maintain sterility. We have provided a perspex “tent” that helps prevent contamination from airborne contaminants. Before use, swab down the inside surface with ethanol-soaked cotton wool. Sterile Petri dishes should only be opened under the tent covering. Forceps/tweezers can be cleaned similarly using an ethanol-soaked swab.
5. You have have been provided additional sterile agar plates containing the standard growth medium (0.5x Gamborg B5 with added vitamins in 1.2% w/v agar) - with a varied composition of added phytohormones. There are 2x control plates without any phytohormone additives, 1x +auxin, 1x +cytokinin, 1x +(auxin & cytokinin). In addition, you will have access to syringes loaded with phytohormone-laden lanolin. (These allow the placement of biocompatible beads of lanolin adjacent to plant material, that can act as artificial sources of phytohormone.
6. If you wish to weigh sterile plant material being transferred, measure the weight of the destination Petri dish before and after transfer. You can weigh the material directly in Week 2 after transfer to a plastic weigh boat, as there will no requirement to maintain sterility then.
7. Sketch out the design of your experiment - your demonstrators are there to help if needed.
8. Implement your plans. Note the state of the plant tissues, before they are left to grow for another week. (You may want to capture images on your phone). 9. Label your plant tissue culture plates. They will be incubated for a week at 22 degrees C, under continual light.
1. You are provided with an agar plate containing Marchantia gemmae that have been growing in sterile media for about one week.
2. Inspect the gemmae in the sealed plate, using a compound microscope and low power (4x) objective. Identify key features on the gemmae: meristematic regions, central portion, rhizoids, oil cells (if present), air pores and chambers.
3. What are the main recognisable features (and possible functional domains) of the gemmae? Auxin has been long implicated in establishment of polarity and cell fate in higher plants. Cytokinins have been implicated in meristem maintenance and antagonism to auxin. How might you disrupt interactions across the young plantlets? Do you have any candidate roles for auxin and cytokinin? What might you look for, if you disrupt and re-plate gemmae? Can you probe the role of auxin and cytokinin in cell proliferation versus patterning and differentiation?
4. The gemmae are maintained in sterile culture, so any manipulations, including transfer to new plates, should maintain sterility. We have provided a perspex “tent” that helps prevent contamination from airborne contaminants. Before use, swab down the inside surface with ethanol-soaked cotton wool. Sterile Petri dishes should only be opened under the tent covering. Forceps/tweezers can be cleaned similarly using an ethanol-soaked swab.
5. You have have been provided additional sterile agar plates containing the standard growth medium (0.5x Gamborg B5 with added vitamins in 1.2% w/v agar) - with a varied composition of added phytohormones. There are 2x control plates without any phytohormone additives, 1x +auxin, 1x +cytokinin, 1x +(auxin & cytokinin). In addition, you will have access to syringes loaded with phytohormone-laden lanolin. (These allow the placement of biocompatible beads of lanolin adjacent to plant material, that can act as artificial sources of phytohormone.
6. If you wish to weigh sterile plant material being transferred, measure the weight of the destination Petri dish before and after transfer. You can weigh the material directly in Week 2 after transfer to a plastic weigh boat, as there will no requirement to maintain sterility then.
7. Sketch out the design of your experiment - your demonstrators are there to help if needed.
8. Implement your plans. Note the state of the plant tissues, before they are left to grow for another week. (You may want to capture images on your phone). 9. Label your plant tissue culture plates. They will be incubated for a week at 22 degrees C, under continual light.